Context
The optimization of a solar farm system in Egypt, aimed at providing electricity to 50 homes while minimizing investment and maintenance costs, entails a multi-faceted approach. The system is dissected into three subsystems: layout optimization, battery energy storage system (BESS) optimization, and cooling system optimization. Each subsystem is tasked with maximizing efficiency within its domain while adhering to global constraints and requirements.
Interdependencies among the subsystems necessitate a holistic approach. Layout optimization seeks to strategically position solar panels to maximize energy production while minimizing land and panel costs. BESS optimization focuses on efficiently storing surplus energy for later use, ensuring a continuous power supply even during periods of low solar generation. The cooling system optimization aims to enhance panel efficiency by minimizing heat flux through meticulous design considerations.
Financial considerations are paramount, requiring a delicate balance between cost-effectiveness and system performance. The objective is to achieve maximum efficiency across all subsystems while maintaining profitability compared to alternative energy sources. By addressing these complexities and optimizing each subsystem individually, the solar farm system can effectively meet the energy needs of Egyptian communities, contributing to sustainable development and mitigating the impacts of climate change.
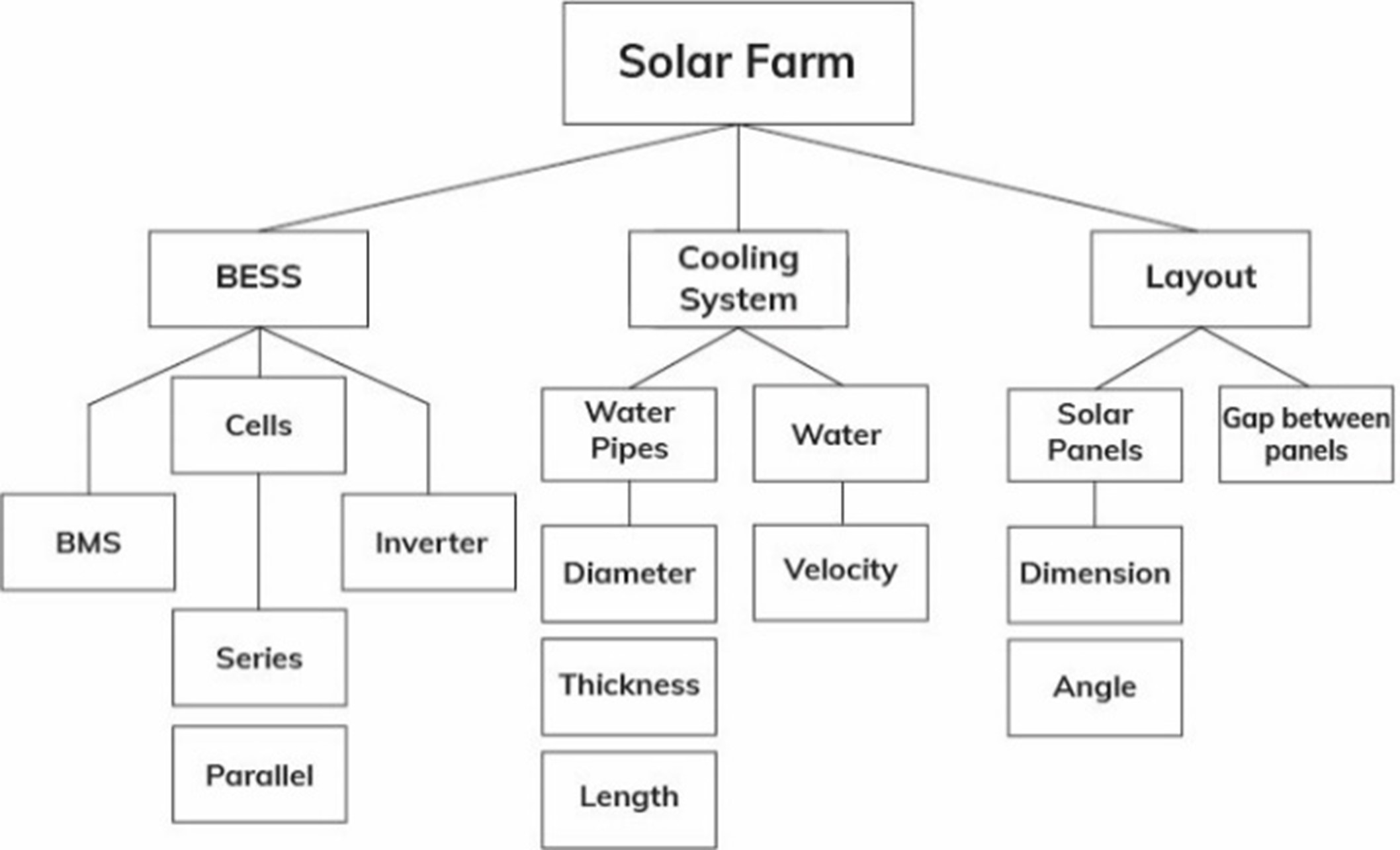
Battery Energy Storage System
In the pursuit of sustainable energy, Battery Energy Storage Systems (BESS) are pivotal in solar installations, storing excess energy for times when the sun isn’t shining, ensuring continuity of supply, especially during peak demand periods. The BESS optimizes efficiency, acting as the heart of a solar farm, balancing the delicate equation of cost and utility to maximize profit over the battery’s lifecycle without sacrificing performance. This optimization involves intricate mathematical modelling considering series/parallel cells, discharge depth, and overall system costs, including installation and inverters.
Design variables like cell numbers and discharge depth, constrained by factors such as voltage range and economic viability against grid costs, are carefully considered. Advanced optimization techniques such as genetic algorithms and Sequential Quadratic Programming (SQP) navigate non-linear constraints, yielding an optimal BESS configuration promising a 23% profit margin, amounting to $24,753 over its lifecycle. This optimized BESS showcases solar farms' potential in offering reliable, profitable energy solutions, meeting community energy demands while contributing to a sustainable future.